Have Questions About
Mycotoxins Testing?
We've Got Answers.
Mycotoxins are toxic compounds produced by certain molds that can contaminate crops and food products, posing significant health risks to both humans and animals.
This FAQ section covers much of what you need to know about mycotoxins: from general information about mycotoxins, to how they are regulated and controlled, as well as the various methods used for testing. Understanding these aspects is crucial for ensuring food and feed safety.
Why Choose Romer Labs?
With over 40 years of experience in mycotoxin detection and an extensive portfolio of mycotoxin testing solutions, including state-of-the-art test kits, reference materials, clean-up columns and analytical services, we're here to support food and feed producers in navigating the complexities of mycotoxin management.
Find the Answers You Need
Browse our FAQ section to get insights into mycotoxin-related topics. Can't find what you're looking for? Submit your question today, and let our experts guide you through the intricacies of mycotoxin testing.
Can't Find What You're Looking For?
Mycotoxins are toxic secondary metabolites produced by molds. There are hundreds of mycotoxins, some of them are used as antibiotics (penicillin), others are dangerous to human and animal health (e.g. aflatoxins) when ingested or inhaled. Mycotoxins are often found in agricultural commodities (corn, wheat, barley, nuts, spices, dried fruits, apples, coffee beans etc.).
Secondary metabolites are natural products produced mainly by bacteria, fungi, plants, and algae that are not essential to the organism or directly involved in the growth, development, and reproduction of the organism. They have various functions that can provide advantages to the organism producing them such as defense mechanism, attraction, competition or signaling.
There are hundreds of mycotoxins known to science, produced by various species of fungi. However, in food and feed safety, only a subset of these are regularly tested due to their prevalence and the severity of their health impacts. The most studied and significant mycotoxins in terms of food safety and human and animal health are:
- Aflatoxins (produced by Aspergillus species)
- Ochratoxin A (produced by Aspergillus and Penicillium species)
- Fumonisins (produced by Fusarium species)
- Zearalenone (produced by Fusarium species)
- Trichothecenes (including deoxynivalenol, nivalenol, T-2 toxin, and HT-2 toxin, produced by Fusarium species)
- Patulin (produced by Penicillium, Aspergillus, and Byssochlamys species)
- Citrinin (produced by Penicillium and Aspergillus species)
- Ergot Alkaloids (produced by Claviceps species)
While these are among the most significant, it's important to note that other mycotoxins can also pose risks, depending on the region, climate, and types of crops or feeds involved. Routine testing typically focuses on these key mycotoxins because of their known effects on health, their regulatory importance, and their prevalence in contaminated food and feed products.
Some less commonly discussed but still potentially harmful mycotoxins include:
- Alternariol and Alternariol Monomethyl Ether (produced by Alternaria species): These toxins are often found in fruits, vegetables, and grains. They have been studied for their genotoxic and mutagenic properties.
- Sterigmatocystin (produced by Aspergillus species): A precursor to aflatoxin, sterigmatocystin is carcinogenic and is found in various grains and other plant products.
- Enniatins (produced by Fusarium species): These are cyclic hexadepsipeptides that can have antibiotic properties but are also considered toxic and have been found in cereals and cereal-based products.
- Moniliformin (produced by Fusarium species): Found mainly in maize and other grains, moniliformin is known for its acute toxicity, particularly affecting the cardiovascular system.
- Cyclopiazonic Acid (produced by Aspergillus and Penicillium species): This toxin is often associated with contaminated cheese and other dairy products, as well as grains and nuts. It is a neurotoxin that can cause liver and kidney damage.
- Penicillic Acid (produced by Penicillium and Aspergillus species): This mycotoxin is found in various moldy foods, including grains and nuts, and is known for its potential carcinogenicity.
- Gliotoxin (produced by Aspergillus species): Known for its immunosuppressive properties, gliotoxin is often studied in the context of its role in infections, particularly in immunocompromised individuals.
- Roquefortine C (produced by Penicillium species): Commonly associated with blue cheese, this toxin can be neurotoxic, and although it is usually found in low concentrations in foods, it is a point of concern in moldy products.
Mycotoxins are toxic compounds produced by certain types of fungi that can contaminate food and feed products, posing significant health risks to humans and animals. Several factors make mycotoxins dangerous:
- Toxicity: Mycotoxins can cause acute toxic symptoms like vomiting, diarrhea, and abdominal pain. Chronic exposure can lead to liver and kidney damage, immune suppression, and increased cancer risk. Some mycotoxins may also affect the nervous system, hormonal balance, and reproductive system.
- Stability and Persistence: Mycotoxins are highly stable and can withstand food processing methods, making them difficult to eliminate from contaminated food products.
- Prevalence: Mycotoxins can contaminate a wide range of agricultural products, including grains, nuts, spices, fruits, and animal feeds. They can enter the food chain at multiple points, from crop production and harvesting to storage and processing.
- Impact on Food Safety and Economy:Contaminated food can lead to foodborne illnesses and outbreaks. Economic losses due to crop and product rejection, decreased animal productivity, and the cost of testing and mitigation measures.
- Regulatory Challenges: Detection and regulation of mycotoxins require advanced analytical methods and consistent monitoring, which can be resource-intensive.
- Environmental Factors: Climate change and agricultural practices can influence fungal growth and mycotoxin production, potentially increasing the risk of contamination. Given these factors, managing mycotoxin contamination involves stringent agricultural practices, regular monitoring, and advanced food safety protocols to minimize exposure and protect public health.
Mycotoxins can have a wide range of adverse effects on both humans and animals, depending on the type of mycotoxin, the level and duration of exposure, and the susceptibility of the individual or species:
Acute Toxicity:
- Nausea and Vomiting: Immediate reactions can include gastrointestinal distress, such as nausea, vomiting, and abdominal pain.
- Diarrhea: Some mycotoxins can cause severe diarrhea.
- Respiratory Issues: Inhalation of mycotoxins can lead to respiratory problems, including coughing, wheezing, and difficulty breathing.
- Reduced Feed Intake: Animals exposed to mycotoxins often exhibit reduced appetite and feed refusal.
- Neurological Symptoms: Some mycotoxins can cause tremors, seizures, and other neurological symptoms.
Chronic Toxicity:
- Cancer: Some mycotoxins (e.g. aflatoxins) are potent carcinogens and can increase the risk of liver cancer.
- Immune Suppression: Mycotoxins (e.g. ochratoxin A and aflatoxins) can weaken the immune system, making individuals more susceptible to infections.
- Kidney and Liver Damage: Mycotoxins (e.g. ochratoxin A and aflatoxins) can cause significant damage to the liver and kidneys over time.
- Neurotoxicity: Mycotoxins (e.g. fumonisins) can affect the nervous system, leading to neurological symptoms and developmental issues.
- Reduced Growth and Weight Gain: Chronic exposure to mycotoxins can lead to poor growth rates and reduced weight gain in livestock.
- Reproductive Issues: Some mycotoxins (e.g. zearalenone) can disrupt hormonal balance and lead to reproductive issues, including infertility, spontaneous abortions and birth defects.
- Immune Suppression: Exposure to mycotoxins may cause weakened immune systems, making the body more susceptible to infections and diseases.
- Skin and Mucous Membrane Irritation: Direct contact with mycotoxins can cause skin irritation, rashes, and dermatitis.
- Reduced Milk and Egg Production: Mycotoxin exposure can result in decreased milk production in dairy cows and reduced egg production in poultry.
The overall impact of mycotoxins on both humans and animals can be severe, leading to significant health issues and economic losses. Preventing and managing mycotoxin contamination in food and feed is crucial to safeguarding health and ensuring food safety.
Crops can get contaminated with molds and mycotoxins through various pathways:
Environmental Conditions:
- Moisture: High humidity and moisture levels due to improper drying and storage of crops create an ideal environment for mold growth and mycotoxin production.
- Temperature: Warm temperatures often favor mold growth. Temperature fluctuations can also contribute to condensation, increasing moisture levels.
- Weather Events: Extreme weather conditions, such as heavy rains, floods, and droughts, can stress crops and make them more susceptible to mold infection.
Pre-Harvest Contamination:
- Infected Seeds and Soil: Molds can be present in the soil or on seeds, leading to infection as the plant grows.
- Insect Damage: Insects can damage crops, creating entry points for molds. Some insects also carry mold spores and spread them to plants.
- Plant Stress: Crops under stress due to nutrient deficiencies, drought, or other factors are more vulnerable to mold infection.
Harvest Practices:
- Delayed Harvest: Delaying the harvest of crops can increase the risk of mold contamination.
- Mechanical Damage: Rough handling during harvest can damage crops, making them more susceptible to mold infection.
Post-Harvest Handling and Storage:
- Improper Drying: Inadequate drying of crops before storage can leave them with high moisture content, leading to mold growth.
- Poor Storage Conditions: Storage facilities that are not properly ventilated, have high humidity, or are not cleaned adequately can facilitate mold contamination.
- Contaminated Storage Facilities: Previous infestations can leave mold spores in storage facilities, which can contaminate new crops.
Processing and Transportation:
- Cross-Contamination: During processing, crops can become contaminated through contact with contaminated equipment or surfaces.
- Inadequate Transportation Conditions: Poor transportation conditions, such as high humidity or temperature fluctuations, can favor mold growth and mycotoxin production during transit.
Biological Factors:
- Natural Microflora: Some molds are naturally present on crops and can produce mycotoxins if conditions become favorable.
- Competitive Microorganisms: The presence of other microorganisms can either inhibit or promote the growth of mycotoxin-producing molds.
Preventing mold and mycotoxin contamination requires careful management of environmental conditions, proper agricultural practices, effective storage solutions, and frequent in-process controls.
Different grains can be contaminated with various mycotoxins, each produced by specific fungi. The contamination of these grains with specific mycotoxins depends on several factors, including the type of fungus present, environmental conditions, and agricultural practices.
Here is an overview of the most common grains and the mycotoxins they are susceptible to:
- Corn (Maize): Aflatoxins, Fumonisins, DON, Zearalenone, Ochratoxin A
- Wheat: DON, Zearalenone, Ochratoxin A, T-2 and HT-2 Toxins
- Barley: DON, Zearalenone, Ochratoxin A, T-2 and HT-2 Toxins
- Oats: DON, Zearalenone, Ochratoxin A, T-2 and HT-2 Toxins
- Rice: Aflatoxins, Ochratoxin A, DON
- Sorghum: Aflatoxins, Fumonisins, DON, Zearalenone
- Rye: DON, Zearalenone, Ochratoxin A, Ergot Alkaloids
Aflatoxin B1 is considered the most dangerous mycotoxin. It is highly toxic at very low levels, carcinogenic, and can cause severe acute and chronic health issues, including liver cancer, in both humans and animals.
Depending on the contamination level all mycotoxins can have severe adverse health effects when ingested.
Additionally, the presence of multiple mycotoxins can lead to synergistic effects, where the combined impact is greater than the sum of their individual effects. This means that co-exposure to different mycotoxins can result in more severe health outcomes, complicating the risk assessment and management of mycotoxin contamination in food and feed.
For example, research has shown that the combination of aflatoxins and fumonisins can result in enhanced liver toxicity and increased cancer risk compared to each mycotoxin alone. When present together, these mycotoxins can interfere with each other's detoxification processes in the body, leading to higher toxicity levels and more severe health impacts. Deoxynivalenol (DON) and zearalenone are often found together in cereal grains and can interact to intensify immune suppression and reproductive toxicity.
Depending on the region different mycotoxins can be present in different kind of crops. The occurrence of mycotoxins varies by region and is closely linked to climate and the specific crops grown in those areas.
Aflatoxins
- Regions: Predominantly found in tropical and subtropical regions, such as Africa, Southeast Asia, and South America.
- Commodities: Maize (corn), peanuts, cottonseed, tree nuts, and certain spices. Aflatoxins can also be found in milk and dairy products due to contaminated feed consumed by dairy animals.
Trichothecenes (including Deoxynivalenol)
- Regions: Found in temperate regions, including North America, Europe, and parts of Asia.
- Commodities: Cereals such as wheat, barley, oats, and maize.
Ochratoxin A
- Regions: Found globally but more common in temperate regions such as Europe and North America.
- Commodities: Cereals (wheat, barley, oats), coffee, dried fruit, wine, grapes, and spices.
Fumonisins
- Regions: Commonly found in regions where maize is a staple crop, such as the Americas, Africa, and parts of Asia.
- Commodities: Maize and maize-based products.
Zearalenone
- Regions: Found worldwide, particularly in temperate climates like Europe, North America, and parts of Asia.
- Commodities: Maize, wheat, barley, oats, and other cereals.
Climate change has a significant impact on the production and distribution of mycotoxins. Higher temperatures can accelerate the growth rates of some fungi. For instance, species like Aspergillus and Fusarium, which produce aflatoxins and fumonisins, thrive in warm weather conditions. Consequently, regions that were previously too cool for certain mycotoxin-producing fungi may become suitable as temperatures rise. Fungal growth and mycotoxin production is also heavily influenced by moisture. Increased humidity and changes in precipitation patterns can create more favorable conditions for mycotoxin production. Extreme weather conditions like droughts followed by storms, heavy rains and flooding can also stress and/or damage plants, making them more susceptible to fungal infections.
Aflatoxin is a type of mycotoxin produced by certain species of Aspergillus fungi, particularly Aspergillus flavus and Aspergillus parasiticus. These fungi can contaminate a variety of crops, especially those stored in warm and humid conditions. The most common and concerning crops affected by aflatoxin include peanuts, maize (corn), tree nuts, and some spices.
There are several types of aflatoxins, but the most significant in terms of toxicity and frequency of occurrence are aflatoxins B1, B2, G1, and G2. Among these, aflatoxin B1 is the most toxic and is a potent carcinogen, particularly linked to liver cancer in humans and animals. Aflatoxin exposure can occur through the ingestion of contaminated food, but it can also enter the body via inhalation or skin contact with contaminated dusts or materials.
The effects of aflatoxin on health can vary depending on the level and duration of exposure. Acute aflatoxin poisoning (aflatoxicosis) can lead to liver damage, immune system suppression, and in severe cases, death. Chronic exposure, even at lower levels, is associated with a higher risk of liver cancer and other long-term health issues.
Because of its severe health impacts, aflatoxin levels in food and feed are tightly regulated in many countries. Monitoring and controlling aflatoxin contamination is a critical aspect of food safety, particularly in regions where conditions favor the growth of Aspergillus fungi.
For more information, visit https://www.romerlabs.com/en/library/knowledge/detail/what-is-aflatoxin
Deoxynivalenol (DON), also known as vomitoxin, is a type of mycotoxin produced by certain species of the Fusarium fungus, most notably Fusarium graminearum and Fusarium culmorum. These fungi typically contaminate cereal crops such as wheat, barley, oats, maize (corn), and rye, especially in regions with cool and wet growing conditions.
Deoxynivalenol is part of a larger group of mycotoxins known as trichothecenes, which are known for their ability to inhibit protein synthesis in cells, leading to various toxic effects. The most notable symptoms of DON exposure in humans and animals include nausea, vomiting, diarrhea, abdominal pain, and, in severe cases, immune system suppression. This is why it is often referred to as "vomitoxin."
Although DON is less acutely toxic than some other mycotoxins, its presence in food and feed can still cause significant problems, particularly in livestock, where it can lead to reduced feed intake, growth retardation, and lower productivity. For humans, chronic exposure to low levels of DON through contaminated food can potentially lead to gastrointestinal issues and immune suppression.
Due to these health concerns, many countries have established regulatory limits for DON levels in food and feed. Monitoring and controlling DON contamination is essential for maintaining food safety and protecting both human health and the agricultural economy.
For more information, visit https://www.romerlabs.com/en/library/knowledge/detail/what-is-deoxynivalenol
Ochratoxin A (OTA) is a mycotoxin produced by several species of fungi, primarily Aspergillus ochraceus and Penicillium verrucosum. These fungi can contaminate a wide range of food products, including cereals (such as wheat, barley, and oats), coffee, dried fruits, wine, grape juice, and certain spices. The contamination typically occurs during storage, especially under conditions of high humidity and temperature.
Ochratoxin A is particularly concerning due to its nephrotoxic effects, meaning it can cause damage to the kidneys. In addition to nephrotoxicity, OTA has been shown to be carcinogenic, immunosuppressive, and teratogenic (causing developmental defects). Chronic exposure to low levels of OTA is linked to a higher risk of kidney disease, including a condition known as Balkan endemic nephropathy, which is prevalent in certain regions of Europe.
Ochratoxin A can accumulate in the food chain, particularly in animal products like meat and milk, when livestock consume contaminated feed. This potential for bioaccumulation raises additional food safety concerns.
Given its health risks, regulatory bodies in many countries have established limits on the allowable levels of OTA in food and feed. Monitoring and controlling ochratoxin A contamination is a key component of food safety programs, particularly in industries dealing with at-risk products like cereals, coffee, wine, and dried fruits.
For more information, visit https://www.romerlabs.com/en/library/knowledge/detail/what-is-ochratoxin
Zearalenone (ZEA) is a mycotoxin produced by various species of Fusarium fungi, most notably Fusarium graminearum, Fusarium culmorum, and Fusarium crookwellense. These fungi commonly infect cereal crops such as maize (corn), wheat, barley, oats, and sorghum, particularly under cool, wet conditions during the growing and harvest seasons.
Zearalenone is known for its estrogenic effects, meaning it can mimic the hormone estrogen in animals and humans. This can lead to reproductive issues, particularly in livestock, where exposure to zearalenone-contaminated feed can cause symptoms such as infertility, reduced fertility rates, swelling of the reproductive organs, and abnormal reproductive cycles. Pigs are especially sensitive to the effects of zearalenone, but other animals, including cattle and poultry, can also be affected.
While zearalenone is less acutely toxic than some other mycotoxins, its hormone-like activity poses significant risks, especially with chronic exposure. In humans, the potential health effects are still being studied, but there is concern about the long-term impact of consuming food products contaminated with zearalenone, particularly in regions where exposure levels are higher.
To protect both animal and human health, many countries have established regulatory limits on the allowable levels of zearalenone in food and feed. Monitoring for zearalenone contamination is especially important in regions prone to Fusarium infections, as well as in industries that process cereals and grains.
For more information, visit https://www.romerlabs.com/en/library/knowledge/detail/what-is-zearalenone
Fumonisins are a group of mycotoxins primarily produced by Fusarium verticillioides and Fusarium proliferatum, fungi that commonly infect maize (corn) and other cereal crops. The most prevalent and toxic of the fumonisins is fumonisin B1 (FB1), which is of significant concern due to its impact on both human and animal health.
Fumonisins are associated with several adverse health effects:
- Human Health: Chronic exposure to fumonisins, particularly through the consumption of contaminated maize, has been linked to esophageal cancer and liver cancer in humans. Additionally, fumonisins have been implicated in neural tube defects, which are serious birth defects of the brain and spine, in populations with high maize consumption.
- Animal Health: In animals, fumonisins can cause a range of toxic effects, depending on the species. In horses, fumonisins can lead to equine leukoencephalomalacia (ELEM), a fatal neurological disorder. In pigs, fumonisins can cause pulmonary edema (fluid accumulation in the lungs), leading to respiratory distress and potentially death. Other livestock, including poultry, can also suffer from reduced growth rates and impaired health due to fumonisin exposure.
Fumonisins disrupt the synthesis of sphingolipids, essential components of cell membranes, which contributes to their toxicity. Given the severe health risks associated with fumonisins, many countries have established strict regulatory limits on fumonisin levels in food and animal feed.
Monitoring and controlling fumonisin contamination is a critical aspect of food safety, particularly in regions where maize is a staple food. Proper storage, crop rotation, and other agricultural practices can help reduce the risk of fumonisin contamination.
For more information, visit https://www.romerlabs.com/en/library/knowledge/detail/what-is-fumonisin
T-2 and HT-2 toxins are closely related mycotoxins belonging to the trichothecene family, produced by certain species of Fusarium fungi, particularly Fusarium sporotrichioides and Fusarium langsethiae. These toxins can contaminate various cereal grains, including wheat, barley, oats, maize, and rye, especially in cool and moist growing conditions.
Toxicity and Health Effects:
- Human Health: T-2 toxin is one of the most toxic trichothecenes, and exposure can lead to a wide range of severe health effects. These include skin irritation, blistering, and necrosis, as well as gastrointestinal distress, immune system suppression, and bone marrow damage, leading to decreased blood cell production. HT-2 toxin, a metabolite of T-2, shares similar toxic properties but is generally considered slightly less potent. Acute exposure to T-2 toxin has been linked to alimentary toxic aleukia (ATA), a condition marked by severe gastrointestinal symptoms, bone marrow damage, and immune system failure. Chronic exposure may increase the risk of cancers and other serious health issues.
- Animal Health: In livestock, particularly pigs and poultry, T-2 and HT-2 toxins can cause reduced feed intake, poor growth, immune suppression, and lesions in the gastrointestinal tract. Chronic exposure in animals can lead to reproductive problems and a heightened susceptibility to infections.
Given their high toxicity, many countries have set regulatory limits for T-2 and HT-2 toxins in food and feed, especially in cereals and cereal-based products. Monitoring and controlling these toxins is crucial to ensuring food safety, particularly in areas where Fusarium contamination is common.
To reduce the risk of contamination, effective agricultural practices such as crop rotation, proper storage, and minimizing crop damage are essential. Additionally, decontamination techniques are often employed to lower toxin levels in food and feed when contamination occurs.
For more information, visit https://www.romerlabs.com/en/library/knowledge/detail/what-is-t-2ht-2
Citrinin is a mycotoxin produced by several species of fungi, most notably Penicillium citrinum, Penicillium verrucosum, and Aspergillus species. This mycotoxin was first isolated from Penicillium citrinum and is commonly found in stored grains, including rice, wheat, barley, and maize, as well as in some fermented foods and certain types of cheese.
Toxicity and Health Effects:
- Human Health: Citrinin is primarily known for its nephrotoxic effects, meaning it can cause damage to the kidneys. While it is less potent than some other mycotoxins, chronic exposure to citrinin can still pose significant health risks, particularly in populations that consume large amounts of contaminated grains or fermented products. There is also some evidence suggesting that citrinin may have genotoxic effects, meaning it could potentially damage DNA and contribute to cancer risk, although more research is needed in this area.
- Animal Health: In animals, citrinin exposure can lead to kidney damage, reduced growth rates, and reproductive issues. Livestock consuming contaminated feed may exhibit symptoms of nephropathy, characterized by kidney dysfunction, which can impact overall health and productivity.
Due to its potential health risks, citrinin levels in food and feed are monitored and regulated in many countries. The focus is particularly on products like stored grains and fermented foods, where citrinin contamination is most likely to occur.
To prevent citrinin contamination, proper storage conditions for grains and food products are essential, as the growth of citrinin-producing fungi is favored by warm and humid environments. Additionally, agricultural practices that minimize fungal growth during the growing and harvesting stages can help reduce the risk of citrinin contamination.
For more information, visit https://www.romerlabs.com/en/library/knowledge/detail/what-is-citrinin
Patulin is a mycotoxin produced by several species of fungi, most notably Penicillium expansum, Aspergillus, and Byssochlamys. This mycotoxin is most commonly associated with moldy fruits, particularly apples and apple products such as juice and cider, but it can also be found in other fruits like pears and grapes.
Toxicity and Health Effects:
- Human Health: Patulin is known for its potential to cause acute toxic effects, particularly affecting the gastrointestinal system. Symptoms of patulin exposure include nausea, vomiting, and gastrointestinal disturbances. While patulin is considered less potent than some other mycotoxins, it is still a concern due to its potential genotoxic effects, meaning it can damage DNA and possibly contribute to cancer development. There is also evidence that patulin may have immunosuppressive properties, which could compromise the body’s ability to fight infections.
- Animal Health: In animals, patulin exposure can lead to similar gastrointestinal issues, as well as immune system suppression. However, the levels of patulin typically found in contaminated food are more concerning for human health due to the lower body weight and different consumption patterns in humans compared to animals.
Due to the potential health risks associated with patulin, many countries have established regulatory limits on its levels in food products, particularly apple juice and other apple-derived products. These regulations are aimed at minimizing exposure, especially in children, who are more vulnerable to the effects of this mycotoxin.
Preventing patulin contamination involves careful monitoring of fruit quality during harvest and processing. Damaged or moldy fruits are more likely to harbor Penicillium expansum and other fungi that produce patulin, so removing these from the production line is essential. Proper storage and handling of fruits can also help reduce the risk of patulin contamination in food products.
For more information, visit https://www.romerlabs.com/en/library/knowledge/detail/what-is-patulin
Various organizations have listed maximum levels for mycotoxins in food and feed. The most detailed and strictest being the ones set by the European Union (EU). The EU has published various regulations and recommendations for many matrix-toxin combinations applicable in the EU and for goods that are imported into the EU. Regulated toxins are aflatoxins (total, B1 and M1), deoxynivalenol, fumonisins (B1 and B2), ochratoxin A, patulin, zearalenone, citrinin, T-2/HT-2 toxin and ergot alkaloids.
In the US the Food and Drug Administration (FDA) sets guidance levels of mycotoxins in food and feed products in certain commodities (aflatoxins, deoxynivalenol, fumonisins, ochratoxin A, zearalenone and patulin).
The Codex Alimentarius, an international guideline established by the Food and Agricultural Organization (FDA) and the World Health Organization (WHO) sets international food standards and provides maximum levels for aflatoxins total, aflatoxin M1, deoxynivalenol, fumonisins, ochratoxin A and patulin.
Mycotoxin regulations in Asia vary by country, but many Asian countries align their mycotoxin regulations with international standards set by Codex Alimentarius Commission, ensuring consistency in food safety practices. To name a few examples, national regulations and guidelines in China are set by the General Administration of Quality Supervision, Inspection and Quarantine (AQSIQ) and the China Food and Drug Administration (CFDA). Regulations related to food and feed safety in Japan are overseen by the Ministry of Health, Labour and Welfare (MHLW) and the Food Safety and Standards Authority of India (FSSAI) is responsible in India. There is also the Association of Southeast Asian Nations (ASEAN) harmonizing standards and guidelines in for this region.
Different mycotoxins have varying levels at which they become hazardous to humans and animals if ingested. Regulatory agencies have established different maximum levels and guidance levels for various mycotoxins to ensure safety.
The European Union has set the strictest regulations/guidelines worldwide. Thresholds for aflatoxins (total, B1 and M1), deoxynivalenol, fumonisins (B1 and B2), ochratoxin A, patulin, zearalenone, citrinin, T-2/HT-2 toxin and ergot alkaloids in food and feed have been established.
Other countries/regions have different maximum levels and it is crucial to consult the regulations for your region. Regulatory agencies such as the FDA, EFSA (European Food Safety Authority), and other national food safety authorities provide maximum levels/guidance levels as well as guidelines handling contaminated goods.
These regulatory limits are frequently reviewed and updated based on new scientific data.
For more information, visit https://www.romerlabs.com/en/library/knowledge/detail/new-mycotoxin-eu-regulations-key-changes-and-updates
Mycotoxins can be reduced with various strategies, both pre- and post-harvest:
- Seasonable harvesting to prevent fungal growth on crops
- Timely drying of crops after harvest to quickly reduce the moisture of the crop and prevent fungal growth and mycotoxin production
- Proper storage management: clean storage facilities, low humidity during storage, regular inspection of stored goods
- Reduction of mycotoxins in contaminated crops:
- Mechanical/physical sorting
- Enzymes to bind/degrade mycotoxins
- Apply fungicides or chemicals on contaminated crops to decontaminate the crops
Mycotoxin rapid tests, such as LFDs (Lateral Flow Devices) and ELISAs (enzyme-linked immunosorbent assays), are valuable tools for mycotoxin screening, providing quick results that are suitable for on-site testing and routine monitoring.
However, the accuracy of these tests can be influenced by several factors, contributing to the total uncertainty of mycotoxin testing.
Factors Affecting Accuracy:
- Sampling: Mycotoxins are often unevenly distributed within a lot, so obtaining a representative sample is crucial for obtaining results that accurately reflect the mycotoxin concentration of the entire batch. In fact, sampling is one of the most significant sources of variability, contributing up to 88% of the total uncertainty in mycotoxin testing.
- Sample Preparation: The efficiency with which mycotoxins are extracted from the sample during preparation can significantly impact the results. Inadequate or inconsistent extraction procedures can lead to underestimation or overestimation of mycotoxin levels.
- Analysis: The analytical error, which occurs during the actual testing process (e.g., in LFD, ELISA, or HPLC analysis), is typically smaller compared to sampling and sample preparation errors. Modern analytical methods are generally precise and have been well-validated, meaning that the variability introduced during the analytical phase is often less significant. However, analytical errors can still occur, particularly if the test is not properly calibrated or if the method used is not suitable for the specific matrix or mycotoxin being analyzed.
Proper sampling techniques (use of representative and well-prepared samples), consistent test protocols (standardized testing protocols and manufacturer instructions), and confirmation of results with laboratory-based methods are crucial for reliable mycotoxin detection and management.
No single method can be considered the gold standard for all situations. ELISA and LFDs (Lateral Flow Devices) are widely used for their speed and cost-effectiveness. Reference Testing (HPLC and LC-MS/MS) is better suited if samples need to be analyzed for multiple mycotoxins at once and in specific cases, such as complex matrices and very low mycotoxin concentrations.
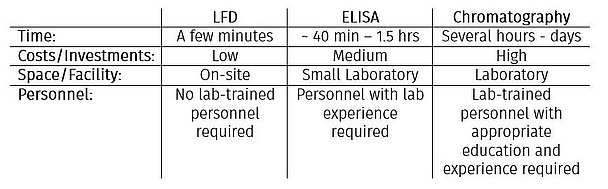
There are several factors that have to be considered before deciding on the most appropriate mycotoxin testing method.
- Time
- Costs/Investment
- Space/Facility
- Personnel
After considering all these factors the individually most appropriate method for mycotoxin testing can be decided upon.
There are various methods to detect and quantify mycotoxins in a sample. These methods are typically categorized into rapid testing and reference testing.
Rapid Testing:
Rapid tests are quick and easy to perform, often providing results within minutes. These tests are widely used on-site by production workers and are crucial for food/feed safety and environmental monitoring due to their convenience and ability to provide timely information.
Common types of rapid tests include:
- LFD (Lateral Flow Device): A quick diagnostic test that provides results through a simple visual indication, often similar to a pregnancy test.
- ELISA (Enzyme-Linked Immunosorbent Assay): A widely used test that utilizes antibodies and color change to identify and quantify mycotoxins in a sample.
Reference Testing:
Reference testing involves standardized, often more accurate and precise methods conducted in a laboratory by trained personnel. These methods are used to obtain validated results, confirm preliminary test results, or provide benchmark data against which other methods or results can be compared.
Common reference testing methods include:
- HPLC (High-Performance Liquid Chromatography): A powerful analytical technique used to separate, identify, and quantify mycotoxins in a sample.
- LC-MS/MS (Liquid Chromatography-Mass Spectrometry/Mass Spectrometry): An advanced method combining liquid chromatography with mass spectrometry to provide highly sensitive and specific mycotoxin analysis.
LFD stands for Lateral Flow Devices. LFDs are simple, rapid diagnostic tools used to detect target analytes, such as mycotoxins. They are primarily used for on-site testing due to their convenience and speed. Performing these tests does not require a laboratory or trained laboratory personnel, making them accessible for quick, on-the-spot testing.
ELISA stands for Enzyme-Linked Immunosorbent Assay. It is a widely used method for detecting and quantifying substances, such as mycotoxins, allergens, and other analytes. ELISA tests are known for their sensitivity and time efficiency, as they allow for the simultaneous analysis of multiple samples.
HPLC and LC-MS/MS are analytical methods used to separate, identify, and quantify components of a mixture in a liquid sample. Both techniques are forms of liquid chromatography. HPLC stands for High-Performance Liquid Chromatography, while LC-MS/MS stands for Liquid Chromatography-Mass Spectrometry/Mass Spectrometry.
In both methods, high pressure is used to push solvents and the liquid sample through a column filled with adsorbent material. Different components of the sample interact differently with the adsorbent material, causing them to exit the column at different times. These components are then identified and quantified by a detector.
Clean-up columns are a crucial component in mycotoxin testing. They are used to purify sample extracts and/or concentrate mycotoxins from complex food and feed sample extracts before analysis. This purification step helps to improve the accuracy, sensitivity and reliability of the subsequent analytical methods, such as HPLC, LC-MS/MS and ELISA.
The following types of clean-up columns can be distinguished:
- Solid-Phase Extraction (SPE) Columns:
SPE columns are widely used for clean-up in mycotoxin analysis. The columns contain sorbent materials that retain unwanted components (=matrix components) in the sample extract while allowing the extracted mycotoxins to pass through. This process effectively cleans up the sample for subsequent quantification, usually using chromatography.
- Immunoaffinity Columns (IAC):
Immunoaffinity columns contain antibodies specific to certain mycotoxins to be analyzed. These antibodies selectively bind the target mycotoxins from the sample extract while allowing unwanted components (matrix components) to pass through. After elution, the bound mycotoxins are released, resulting in a sample extract that contains only the mycotoxins in solution, allowing for interference-free analysis, usually with chromatography.
Immunoaffinity columns are highly specific and effective but are usually more expensive than SPE columns.
Internal standards are substances added to samples in known quantities before analysis. They can be added before, during, or after the extraction process. Internal standards serve as a point of reference to correct matrix effects, thereby improving the accuracy, precision, and reliability of the quantification method. In mycotoxin analysis, internal standards are commonly used for accurate quantification with chromatography, particularly in LC-MS/MS.
An internal standard must have the following characteristics:
- Properties similar to the analyte.
- Close similarities in extraction and derivatization behavior.
- Similar chromatographic behavior as the analyte.
Isotopically labeled standards are widely used as internal standards in mycotoxin testing. These are compounds that are chemically identical to the target analyte but contain stable ¹³C isotopes at specific positions within the molecule.
¹³C isotopically labeled internal standards have identical chemical properties to the analyte of interest, meaning they behave the same during liquid chromatography but can be distinguished during detection by their mass difference. This makes them ideal for correcting matrix effects in LC-MS/MS quantification.
Matrix effects in mycotoxin testing refer to the influence of other components within the sample (the "matrix") on the accuracy and reliability of the test results.
The matrix includes everything in the sample except the mycotoxin being tested for. Matrix effects can interfere with the detection and quantification of mycotoxins, potentially leading to increased variability and inaccurate results, including false positives or false negatives.
The LOD (Limit of Detection) is the lowest number of mycotoxins that can be detected and distinguished from a blank sample, in a defined matrix, by a testing method.
LOQ (Limit of Quantification) is the lowest number of mycotoxins that can be quantitatively measured with acceptable precision and accuracy.
Recovery rate in mycotoxin testing and other analytical methods refers to the percentage of an analyte that is successfully extracted and detected from a sample compared to the known amount/concertation in the sample. It is a measure of the efficiency and accuracy of the extraction and analysis process. The recovery rate (%) is calculated using the formula:
Recovery Rate [%] = (Amount detected / True value) × 100
Various Factors can affect the recovery rate, for example the commodity, extraction efficiency and the analytical method used.
The recovery rate is a critical parameter in analytical quantification of substances, providing information about the accuracy and reliability of the method used. It ensures that the results obtained are valid.
An acceptable recovery rate can vary depending on regulatory guidelines, the specific mycotoxin being tested, the matrix of the sample and the analytical method used.
The FDA's guidance for mycotoxin testing in foods specifies that the recovery of mycotoxins in fortified samples should be between 70% and 120% for methods intended for regulatory purposes. These values are frequently used in mycotoxin testing.
RSD stands for Relative Standard Deviation. It is a statistical measure that expresses the amount of variability or dispersion in a set of data relative to the mean (average) value. RSD is commonly used in analytical chemistry and other scientific fields to assess the precision or reproducibility of measurements. Mathematically, RSD is calculated as follows:
SD [%] = (Standard Deviation / Mean) × 100
A low RSD indicates that the data points are closely clustered around the mean, suggesting good precision. A high RSD means greater variability among the data points, which usually means lower precision or more variability of the measurements.
RSD is widely used in quality control and method validation to evaluate the reliability and consistency of results obtained.
An acceptable RSD in mycotoxin testing can vary depending on regulatory requirements, the specific mycotoxin being analyzed, and the analytical method used. An RSD between 5 and 10 % is usually considered acceptable. Laboratories often aim for and RSD below 10% to demonstrate the reliability and reproducibility of their methods.
Accuracy refers to how close a measurement is to the true value while precision refers to how close measurements of the same item are to each other.
Accuracy and Precision are independent from each other which means it is possible to be precise but not accurate, and it is also possible to be accurate without being precise. Results in mycotoxin testing should be both - accurate and precise!
Mycotoxins are unevenly distributed among contaminated crops, making a correct sampling process crucial to obtaining a sample that accurately represents the whole lot. Multiple samples should be taken from different parts of the lot (top, middle, bottom) to create a composite sample large enough to be representative. Afterward, all samples collected must be combined, thoroughly mixed, and ground before being divided into representative sub-samples for analysis.
Since mycotoxins are regulated at very low concentrations, and the sampling process is critical for accurate analysis results, the European Union has published regulations concerning the correct sampling and analysis of mycotoxins (Commission Implementing Regulation (EU) 2023/2782).
